Lesson 2: Covalent Bonding
Part a: Covalent Bonds
Part 2a: Covalent Bonds
Part 2b:
Lewis Electron Dot Structures
Part 2c:
Double and Triple Bonds
Part 2d:
Octet Breakers
Part 2e:
Formal Charge Considerations
Atomic Interactions
The graph at the right shows the potential energy of two atoms as a function of the distance that separates the two atoms. The looping animation shows the atoms approaching each other from infinite separation to a very close approach. There are three curves on the graph. The dashed orange curve represents the effect of repulsive forces between protons and repulsive forces between electrons. These forces have a destabilizing effect upon the collection of two atoms and increase their energy. The dashed blue curve represents the effect of attractive forces between the protons of one atom and the electrons of the other atom. These forces have a stabilizing effect upon the system and lower its energy. The solid red curve is the net effect of these two forces upon the system. It is the sum of the orange line and the blue line.
There are four locations on the graph that deserve some focused discussion. They have been marked with a letter below.
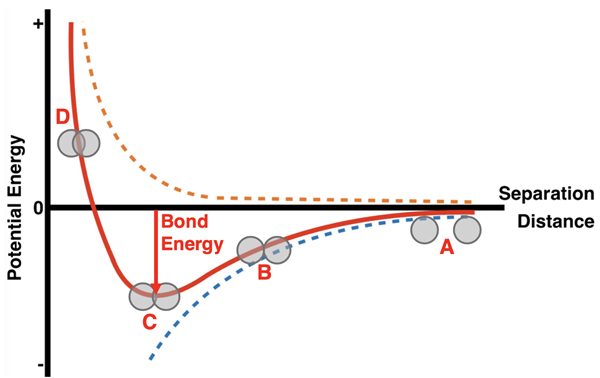
Location A is a point of large separation. By definition, the zero-energy location is a location of infinite distance between the molecules. As the molecules approach from infinite separation, we begin to see the potential energy decreasing slightly. This is a sign of increasing stability. For a location such as A, there’s very little interaction between atoms and very little gain in stability. At location B, there is an obvious lowering of energy and an increase in stability. The atoms are touching, and the attractive interactions dominate any destabilizing repulsions. At location C on the graph, the system of two atoms is at its lowest energy and its greatest stability. The attractive interactions are optimized. The depth of the curve at this location is referred to as the bond energy. It is the amount of energy stored in the bond. As the separation begins to decrease from location C towards location D, the potential energy begins to increase. The repulsive forces between atomic nuclei (protons) becomes increasingly significant. These repulsions cause the stability to decrease. For greatest stability, the two atoms spend most of their time with a separation distance that resembles that of location C.
Stability Secrets
The bond described above is known as a covalent bond. The two atoms are sharing their electrons with one another. The protons of one atom are attracting the electron cloud of the other atom … and vice versa. By sharing their electrons, the atoms are more stable together than if they existed as individual atoms. Is there an overarching principle that can guide our thinking about what makes covalently bonded atoms stable? There is!
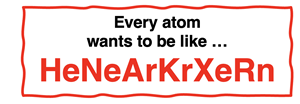
Atoms want to be like HeNeArKrXeRn. This is the overarching principle. Just like ionic bonding discussed in Lesson 1, an atom bonds covalently in order to obtain an octet of electrons in its outermost electron shell. All noble gases have a ns2np6 electron configuration where n is the period number. Helium is the lone exception. It has a 1s2 electron configuration. All s and p orbitals of the outermost electron shell are completely filled with electrons. That is a total of eight valence shell electrons. Noble gases have an octet of electrons in their outermost electron shell. This configuration makes noble gases electronically stable. Atoms of other elements will gain, lose, and share electrons in an effort to obtain an octet of outer shell electrons. This is known as the octet rule. When an atom engages in covalent bonding, it will share electrons in an effort to surround itself by an octet of outer shell electrons. Hydrogen, lithium, and beryllium follow the duet rule in an effort to acquire helium’s electron configuration.
Ionic vs. Covalent Bonding
A fluorine atom has seven valence electrons in its n=2 electron shell. It would like to have an octet – eight electrons. It needs one more electron. It has two options available. The first option is that it could interact with a metal and have an electron transferred to it. The transferred electron is owned by fluorine, and it becomes an ion with a 1- charge. It bonds with the metallic atom that transferred the electron. This is called an ionic bond. Ionic bonds were discussed in detail in Lesson 1.
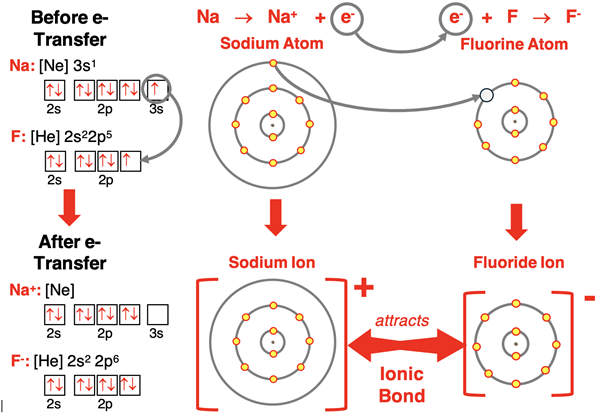
The second option involves approaching a nonmetal (usually) atom that also needs to gain electrons to acquire an octet. While the other atom isn’t willing to transfer an electron, it is willing to share. Both atoms offer an electron to each other and share the pair of electrons. Neither atom owns the electrons. They simply have a pair of electrons that they are sharing. The mathematics of this sharing is simple. Atom A puts one electron forward. Atom B puts one electron forward. That’s two electrons total. Each atom attracts the electron pair. By offering one electron to the shared pair, an atom can increase its total of valence shell electrons by 1. The added electron comes from the other atom. This is illustrated in the diagram below with two fluorine atoms forming a covalent bond.
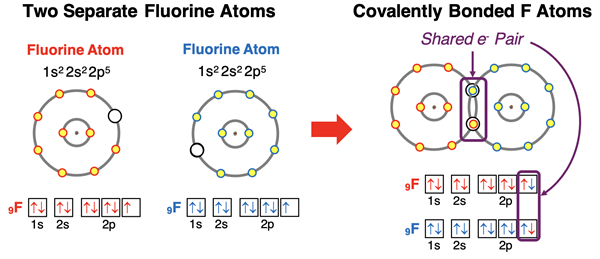
If an atom has less than seven valence shell electrons, then it will have to form more than one covalent bond in order to fill its outer shell with an octet of electrons. Let’s consider oxygen as an example. Oxygen has a 1s22s22p4 electron configuration. It has six valence shell electrons in its outermost electron shell (n=2 shell). It needs two more electrons. It would be expected to form two covalent bonds, acquiring a shared electron from each bond. Suppose it meets up with hydrogen atoms. Hydrogen is a 1s1 element and follows the duet rule. So, two hydrogen atoms will each offer one electron to oxygen. And oxygen will offer 1 electron to each hydrogen for sharing. By sharing two electron pairs with hydrogen, oxygen increases its total valence shell electrons to eight. And each hydrogen atom increases its total valence shell electrons to two, thus satisfying the duet rule.
Polar Covalent Bonds
When electrons are shared among atoms, the shared electrons spend their time in the region between the nuclei of the two atoms. It is the attraction between the electron pair and the nucleus that holds the shared electrons in place. An element’s electronegativity provides a measure of an atoms tendency to pull the shared electrons towards itself. If two atoms have identical electronegativity, then the shared electrons will be shared equally. The electron cloud of the shared pair will be distributed evenly between the two atoms. Such a bond is described as a nonpolar covalent bond. When two nonmetals with significant differences in electronegativity share electrons, the electron cloud will be unevenly distributed. The electrons in the shared pair will spend more time near the more electronegative element. Such a bond is described as a polar covalent bond.
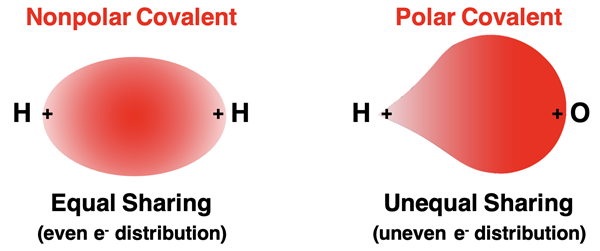
The greater the difference in electronegativity between the covalently bonded atoms, the more that the electron cloud will be unevenly distributed and the more polar that the bond becomes. (Use our Table of Electronegativities to make judgements about the polarity of a bond.)
Bond Polarity
The terms polar, polarized, and polarization are science terms that have been used in fields such as politics. A population of people might be said to be polarized with regards to a particular issue. The use of the term in this context indicates that a group of people have adopted to opposite viewpoints. Polarization is the act of separating into opposites. The term aptly applies to charge within a polar bond.
When a covalent bond is said to be polar, it means there is a separation of opposite charges within the bond. The electron pair is attracted towards the more electronegative atom. In the H-O bond, the electron cloud is pulled away from the hydrogen atom (EN=2.1) and towards the oxygen atom (EN=3.5). Hydrogen becomes the positive dipole and oxygen becomes the negative dipole. We represent this by labeling the hydrogen with a positive partial charge (δ+) and the oxygen with a negative partial charge (δ-). (The δ lower case letter in Greek for delta.) A greater difference in electronegativity would results in a larger partial charge. If the bond were ionic with a transfer of an electron, we would say there is a full +1/-1 charge as opposed to a partial charge.
Dipole Moment Vectors
A polar bond is said to have a dipole moment vector (sometimes referred to as a bond moment). Like any vector, the dipole moment vector has a value or size and a direction. The value or size of the vector depends (among other things) on the difference in electronegativity of the bonded atoms. The direction extends from the positive dipole towards the negative dipole in the direction of the imaginary line connecting the atoms.
Follow these directions to draw a dipole moment vector:
- Use an Electronegativity Table to identify the electronegativity (EN) values of the two atoms.
- Identify the positive dipole (smaller EN value) and the negative dipole (greater EN value).
- Draw an arrow along the bond axis or parallel to it that points to the negative dipole. Place a + sign on the end of the arrow opposite the arrowhead.
Here are three additional examples of dipole moment vectors:
In Lesson 3, we will make a distinction between a dipole moment vector for a bond and a dipole moment vector for a molecule. A molecule may consist of more than one covalent bond. Each bond has a dipole moment vector or bond moment vector. The dipole moment vector for the molecule is the sum of the all the individual dipole moment vectors for each covalent bond. More to come in Lesson 3.
Electron Dot Diagrams
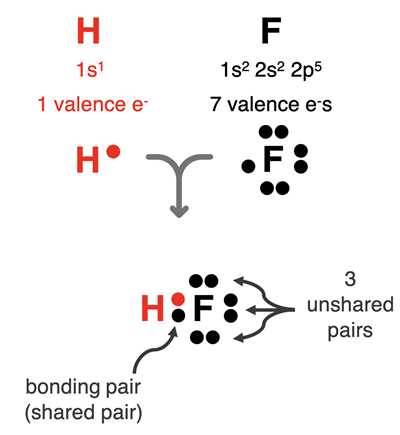
Several conceptual tools from the recent chapter on
Atomic Structure that have carried over into this chapter on
Chemical Bonding and Molecular Shape. Such tools include
electron cloud diagrams,
electron shell diagrams,
orbital box diagrams, and
electron configurations. The most commonly used tool for the remainder of the unit will be the
electron dot diagram. An example for the H-F bond is shown at the right. Hydrogen has one
valence shell electron and would like to have two. Fluorine has seven
valence shell electrons and would like to have eight. Each atom shares an electron with the other atom and a covalent bond is formed. The pair of electrons between the atoms represent the bonding electrons. They are a shared pair of electrons. The other pairs of electrons surrounding fluorine are part of its octet. They are not shared with hydrogen. They are unshared or lone pairs of electrons. This is a simple example of an electron dot diagram for a bond. Observe how the sharing of the electron allows the H atom to satisfy the duet rule and the F atom to satisfy the octet rule.
A second example of an electron dot diagram is shown below.
We will discuss electron dot diagrams in great detail on
the next page of Lesson 2.
Molecular Compounds
Thus far we have seen how a covalent bond can add an additional electron to the total number of
valence shell electrons. You might be wondering (and that’s awesome if you are!) how nitrogen or carbon can acquire an octet of electrons. Nitrogen starts with five outer shell electrons and carbon starts with four outer shell electrons. How can they obtain an octet of electrons? The answer is that these atoms will have to form more than one covalent bond.
The animations below show the formation of compounds containing nitrogen and carbon. Observe the sharing of electrons between the nitrogen and the fluorine atoms and between the carbon and the fluorine atoms. Nitrogen ends up with three shared pairs (or bonding pairs) and one unshared or lone pair. Carbon ends up with four shared pairs and no unshared (lone) pairs.
Electron dot diagrams are incredibly useful tools for depicting how outer shell electrons are used in covalent bonding. They also help us to predict the compounds that are formed as a result of covalent bonding. On
the next page of Lesson 2, we will learn the details about drawing electron dot structures for molecular compounds.
Before You Leave
- Download our Study Card on Covalent Bonding. Save it to a safe location and use it as a review tool. (Coming Soon)
- Practice. Try our Science Reasoning Center activity titled The Periodic Trends. Activities 4 through 5 are great follow-ups to Lesson 2a.
- More great practice ahead with our Bond Polarity Concept Builder. It’s great practice!
- And still more great practice at our Science Reasoning Center with an activity titled The Periodic Table, Elements, and Their Bonds. Activities 2 through 4 are great follow-ups to Lesson 2a.
- The Check Your Understanding section below include questions with answers and explanations. It provides a great chance to self-assess your understanding.
Check Your Understanding
Use the following questions to assess your understanding. Tap the Check Answer buttons when ready.
1. Consider the energy vs. separation distance graph below for two atoms. Curve A (in red) was the curve discussed at the beginning of the page. Which one of the other curves – B (in orange), C (in green), or D (in blue) – represents a bond with a greater bond energy than curve A?
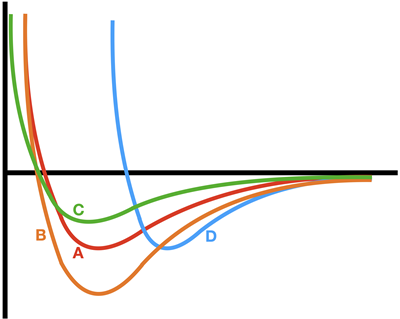
2. Identify the following statements as being true of Ionic Bonds only (IB), Covalent Bonds only (CB), or both types of bonds (BT).
- Occurs primarily between a metal and a nonmetal.
- Electrons are shared between atoms.
- The bond is the result of an electrostatic force of attraction; that is, opposite charges are attracting.
- Occurs primarily between a metal and a nonmetal.
- Involves valence shell electrons.
- Electrons are transferred from one atom to another atom.
- The atoms involved in the bond are attempting to obtain an octet of electrons in their outermost electron shells.
- Partial charges are often formed as the result of the attraction between a nucleus and an electron.
- The bonded atoms are more energetically stable together than they are as individual atoms.
- Involves the attraction of a cation and an anion.
3. Consider the electron shell diagrams for atoms A and B. For each atom, identify the number of valence electrons and the number of covalent bonds the atom could be expected to form with fluorine.
4. Complete the following table:
5. For each of the following pair of elements forming a bond, use an
Electronegativity Table to identify the element with the positive partial charge and the element with the negative partial charge. Then draw the dipole moment vector for the bond.
6. Consider the following three bonds. One is ionic; one is polar covalent; one is nonpolar covalent. Identify which is which.
- H - P
- K - F
- P - Cl
7. Consider the following electron dot structure of oxygen dichloride (OCl
2).
- How many shared electron pairs and how many lone (unshared electron pairs) are on the oxygen atom?
- How many shared electron pairs and how many lone (unshared electron pairs) are on either one of the chlorine atoms?
8. Consider the following electron dot structure of nitrogen tribromide (NBr
3).
- How many shared electron pairs and how many lone (unshared electron pairs) are on the nitrogen atom?
- How many shared electron pairs and how many lone (unshared electron pairs) are on either one of the bromine atoms?