Hold down the T key for 3 seconds to activate the audio accessibility mode, at which point you can click the K key to pause and resume audio. Useful for the Check Your Understanding and See Answers.
Permanent Magnets and Solenoids
The observation that a current-carrying wire experiences a magnetic force when in a magnetic field has led to hundreds of applications. In this lesson, we’ll explore one of these applications—the speaker. We’ll consider the design and operation of a speaker by applying the Force-finding Right Hand Rule. Be it ear buds, headphones, a computer speaker, or the huge loudspeakers that you might experience at a concert, all these work in the same way. As we begin to uncover just how a speaker works, let’s first revisit two types of magnets we’ve already considered in previous lessons: permanent magnets and electromagnets.
Both a permanent magnetic and electromagnet have poles with magnetic field lines that pass through and around the magnet. The electromagnet, however, can be turned 'on' and 'off' whereas the permanent magnet is always 'on'.
A permanent magnet is one where the domains are ‘permanently’ aligned so that the magnetic field is always ‘on.’ An electromagnet is one where the magnetic field can be turned ‘on’ or ‘off’ by allowing current to pass through the coils of a solenoid or by disconnecting the circuit so that no current flows. Electromagnets offer much more versatility. In fact, by changing the direction of the current, we can even change the direction of the magnetic field.
We’ve seen that permanent magnets come in different shapes. Consider, for example, a permanent horseshoe magnet. Let’s put the north pole of the horseshoe magnet inside a solenoid. The image below on the left shows what this might look like. If no current is present in the coils of the solenoid, nothing much happens. What if, however, we allowed current to flow through the coils as shown. A cross-sectional view, the image below, shows the top segments of each coil of the solenoid with current flowing into the page through each loop of wire. We can see that this current is in the horseshoe magnet’s magnetic field. Using the Force-finding Right Hand Rule for currents, we notice that each coil of the solenoid would feel a force to the right.
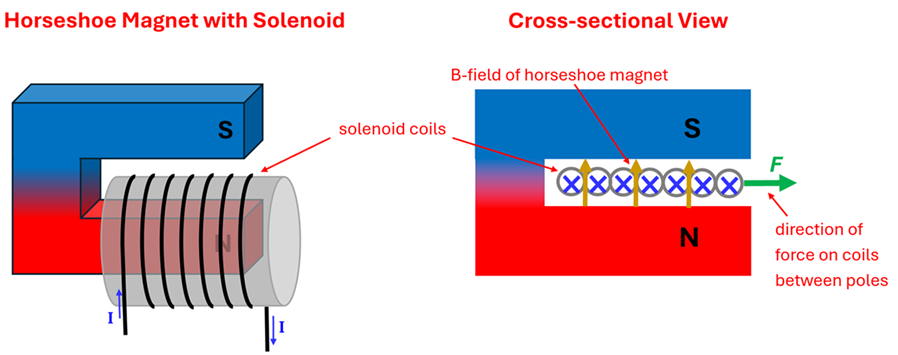
There is another equivalent way to explain this. Recall that, using the Field-finding Right Hand Rule, the solenoid itself creates a magnetic field inside its core due to the current in its coils. In the case we’ve just analyzed, we can see that magnetic field of the solenoid points left and opposes the magnetic field lines that would come out the end of the permanent magnet’s north pole. This is like bringing two north poles together and watching them repel. Whether we use the Force-finding Right Hand Rule to observe the rightward force on the coils or apply the Field-finding Right Hand Rule to the solenoid coils to see that its opposing field would also cause a rightward repelling force on the solenoid, we are can explain this same phenomena in two equivalent ways.
What would happen if we reversed the direction of the current in the solenoid? After all, that is the advantage of using an electromagnet, isn’t it? Let’s use Example 1 to explore this very question.
Example 1: Reversing the Solenoid's Current
Problem: We saw above that the solenoid coils feel a rightward force when current passed through the coils in the direction shown above. In what direction would the solenoid coils feel a force if the direction of current was reversed?
Solution: The permanent horseshoe magnet’s magnetic field will continue to point from north to south—toward the top of the page in our figure. With the current reversed, however, the current through the top segments of each coil is now pointing out of the page. Using the Force-finding Right Hand Rule we see that the force on these coils is now directed to the left. By simply changing the direction of the current we’ve allowed the solenoid to be ‘pulled into’ the horseshoe magnet instead of being repelled by it.
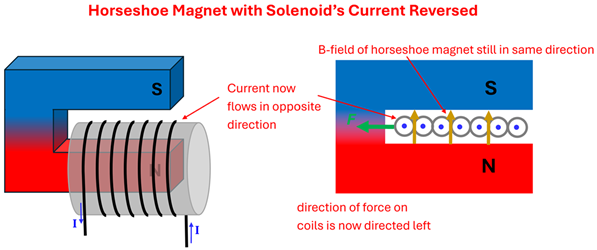
So, by simply reversing the direction of the current we observe the coils of the solenoid feel a force in the opposite direction. This is in essence how a speaker operates! Let’s explore this next.
Anatomy of a Speaker
A speaker can be made using a permanent magnet with one of its poles directed down the center of a solenoid. Since permanent magnets can be formed into different shapes, engineers got clever and essentially wrapped a horseshoe magnet around in a circle. You can visualize this in the images shown below. They then attached a cone—called the speaker diaphragm—to the solenoid so that if the solenoid moves to the left or right, the diaphragm will as well.
This looks very similar to what we just considered above. The only thing new in this situation is that we have a magnetic field that points radially outward (not just upward) from the permanent magnet’s north pole. The cross-sectional view allows us to see that now each segment of wire in the solenoid’s coils will feel a force. Since these forces will all be in the same direction, we’ll get a significant net force—one big enough to move both the solenoid and speaker diaphragm along with it!
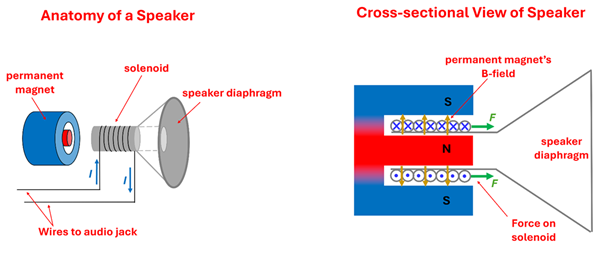
We saw above that when the current in the solenoid was reversed, the solenoid’s coils felt a force that pulled them into the magnet (leftward) instead of being pushed away from the magnet (rightward). So how do we get the current to reverse directions? And how do we get it to change directions so quickly that the speaker diaphragm can actually produce sound? All we have to do is connect the solenoid wires to an audio jack that is plugged into your computer or some other device that has stored audio. Let’s uncover how this works next.
Changing Current Means Changing Force
The audio signal from your computer’s audio jack is merely a complex series of oscillating currents that vary in frequency (how often they change direction) and amplitude (how large the currents are). In fact, all music can be coded in such a signal—or waveform. For the sake of illustration, let’s consider a simple set of sound data recorded as a sine wave. Imagine the current flowing in one direction as it increases from zero to a maximum and then decreases back to zero. This is then followed by the current increasing to a maximum and then decreases to zero but in the opposite direction. If this is repeated, can you see how the electromagnetic force on the solenoid would change directions? Since the speaker diaphragm is connected to the solenoid, it feels this increasing and decreasing force as well. In one case the force is directed to the right which pushes the speaker diaphragm outward. A little later, however, the force is directed to the left, which pulls the speaker diaphragm inward.
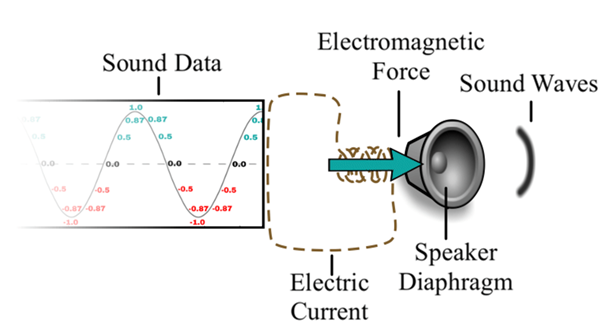
Figure 1 - Click to view Animated version in new tab
Sound data from all music and recorded audio can be saved as a waveform. Many of these waveforms are much more complex than a simple sine wave. Nonetheless, the principle behind how a speaker plays this audio is the same. As the current varies in direction and amount, the speaker’s solenoid and diaphragm respond accordingly.
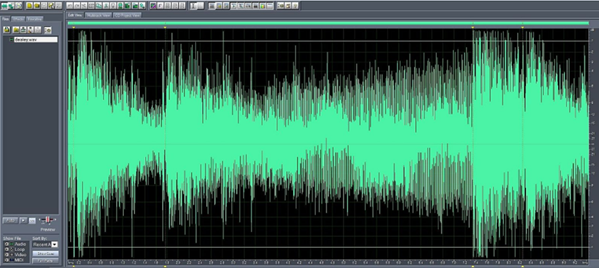
Figure 2
In an upcoming lesson on pressure waves, we will learn that a vibrating object pushes air molecules creating a series of high- and low-pressure areas that oscillate the air between a vibrating object (such as the speaker diaphragm) and our ears. These vibrations then shake our eardrum which sends a signal to our brains where these signals are interpreted as sound. It was simply the force on a current-carrying wire in a magnetic field, however, that allows all this to work!
Are there other practical everyday applications that involve a magnetic force on a current-carrying wire? Indeed, there are. We’ll consider one of these in the next lesson as we explore the physics behind the electric motor!
Check Your Understanding
Use the following questions to assess your understanding. Tap the Check Answer buttons when ready.
1. Determine the direction of the force on the solenoid in each situation below.
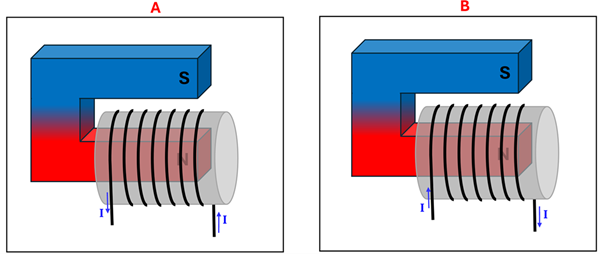
2. True or False. A speaker works because a current-carrying wire feels a force in a magnetic field.
3. True or False. An audio signal can be stored as a waveform that allows varying currents to vary the force on a solenoid in a magnetic field.
4. True or False. If the direction of the magnetic field were reverse instead of the direction of current, the speaker would no longer exert a force on the solenoid.
Figure 1 Source: https://commons.wikimedia.org/wiki/File:Sound_wave_animation.gif
Figure 2 Source: https://commons.wikimedia.org/wiki/File:Adobe_Audition_waveform_of_sound_impulses_on_the_police_dictabelt_analyzed.jpg