Hold down the T key for 3 seconds to activate the audio accessibility mode, at which point you can click the K key to pause and resume audio. Useful for the Check Your Understanding and See Answers.
Lesson 3: Solids
Part a: Crystalline Solids
Part a: Crystalline Solids
Part b:
Amorphous Solids
Part c:
Alloys
What is a Crystalline Solid?
Solids have the unique property of having a fixed shape and a fixed volume. Unlike liquids and gases, if a solid object is moved from one container to another container, neither its shape nor its volume changes. While all solids share this property, two solid objects can be quite different in terms of other properties such as electrical and thermal conductivity, solubility in water, melting points, ductility, and malleability. These differences in properties can be traced to the manner in which the particles or fundamental particles of the solid are arranged and attracted to each other.
Crystalline solids are solids that have a highly organized arrangement of particles. The particles (either atoms, ions, or molecules) of which such solids are composed are arranged into a tightly packed, three-dimensional structure known as a crystal lattice. You can think of the lattice structure as being a large three-dimensional grid. Within this grid, there is a repeating unit, known as the unit cell, that repeats itself throughout the entire structure. Particles occupy the so-called lattice points of the grid. These particles can be atoms, ions, or molecules; we will refer to these particles as the fundamental particle. In a sodium chloride crystal, the fundamental particles are ions - sodium and chloride ions. The entire lattice structure shows these ions occupying the lattice points of the structure.
Because of the orderly arrangement of fundamental particles, naturally occurring crystals exhibit a recognizable appearance. The appearance is often characterized by sharp lines, distinct corners, and geometric patterns. These observable characteristics reflect the orderly arrangement of fundamental particles. Non-crystalline solids or amorphous solids do not display these characteristics. And at the particle level, amorphous solids lack an orderly arrangement of particles. We will discuss amorphous solids in detail on the next page of Lesson 3. Our focus on this page will be crystalline solids.
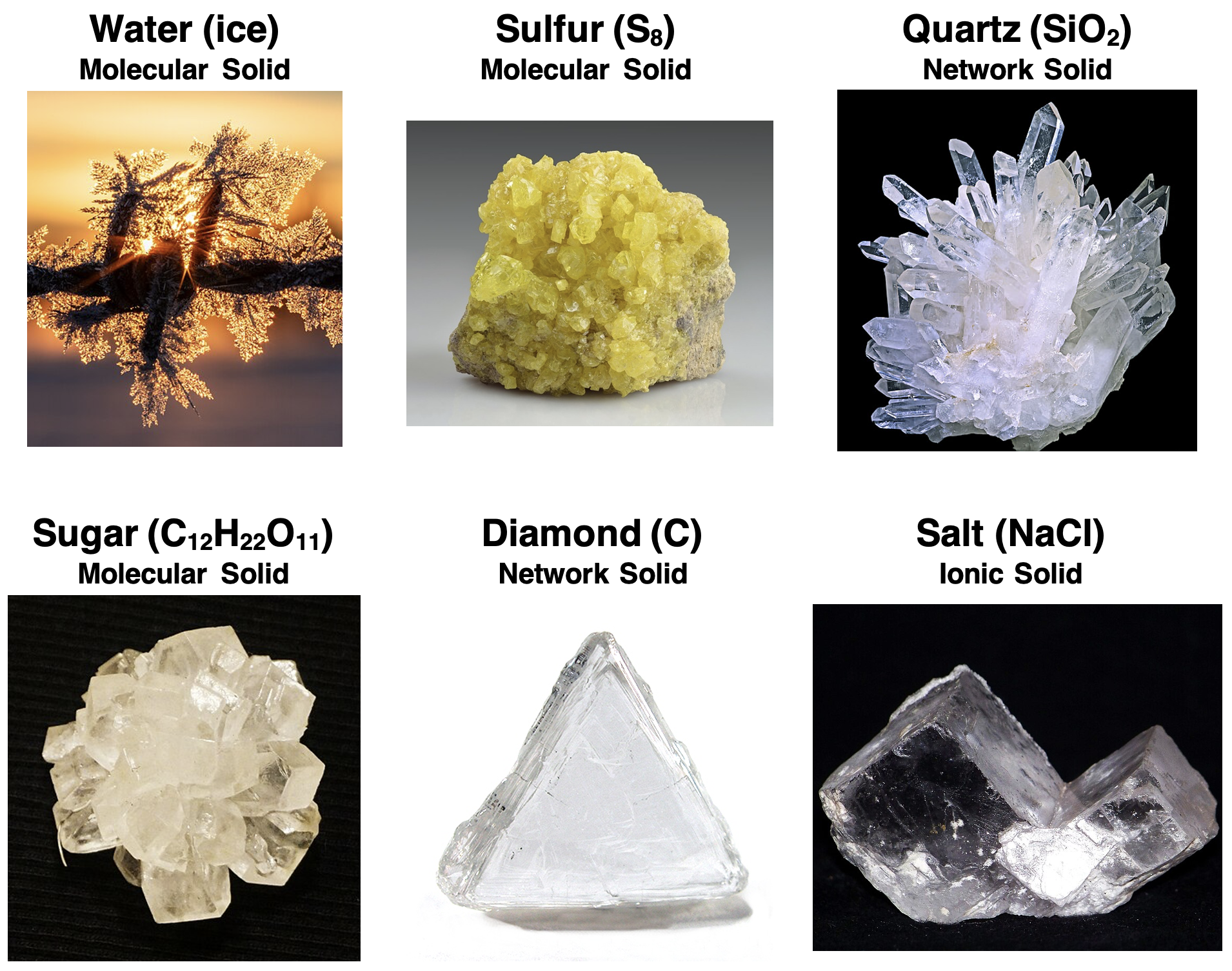
Photo Credits:
Top Left (ice): Wikimedia Commons
Top Center (sulfur): Wikimedia Commons
Top Right (quartz): Wikimedia Commons
Bottom Left (sugar): Wikimedia Commons
Bottom Center (diamond): Wikimedia Commons
Bottom Right (salt): Wikimedia Commons
Types of Crystalline Solids
Let’s consider two common crystalline solids - table salt (sodium chloride, NaCl) and table sugar (sucrose, C12H22O11). At first glance, the two solids may appear very similar. In addition to one tasting salty and the other sweet, their other properties are very different. While they both dissolve in water, sodium chloride produces an aqueous solution that is a strong conductor of electricity. On the other hand, sucrose is a non-electrolyte; solutions of sucrose do not conduct electricity. Sucrose has a relatively low melting point while sodium chloride must be heated to a very high temperature before melting. Sucrose consists of nonmetallic elements that are held together by covalent bonds. Sodium chloride consists of a metal and nonmetal element held together by the stronger ionic bonds.
These differences between sodium chloride and sucrose are a hint of two different types of crystalline solids. Sucrose is classified as a molecular solid. The fundamental particle is a molecule. The molecules are held together by intermolecular forces such as hydrogen bonding, dipole-dipole interactions, and London dispersion forces. Sodium chloride is classified as an ionic solid. The fundamental particle are ions - cations and anions. An inspection of the crystal lattice will not reveal any “sodium chloride molecules”. Rather, the lattice is a vast, repeating grid of sodium and chloride ions held together by very strong ionic bonds.
In addition to molecular and ionic solids, there are also atomic solids. As the name implies, the fundamental particles are atoms. Atoms occupy the lattice position. This type of solid is further broken down into metallic solids and network solids. The fundamental unit of metallic solids are metal atoms. And the fundamental unit of network solids are typically atoms of nonmetal or metalloid elements. These four types of crystalline solids are summarized in the table.
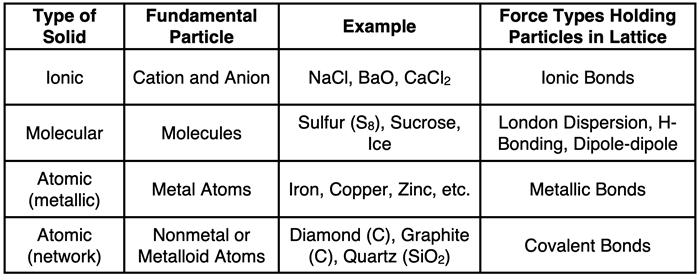
Molecular Solids
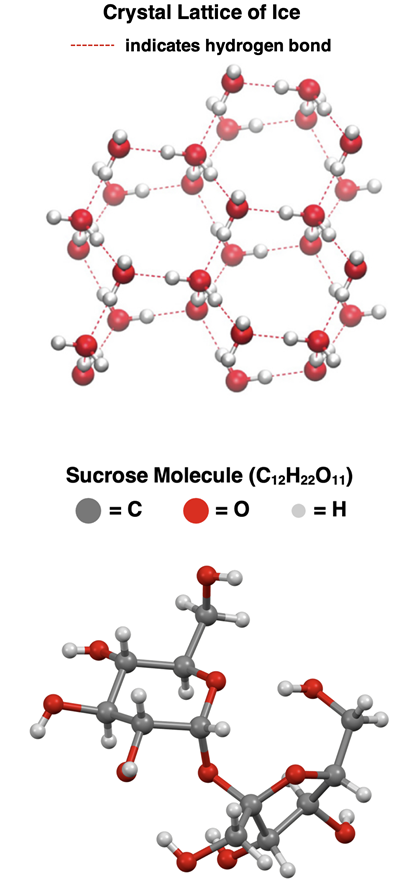
Most covalently bonded molecules are liquids or gases at room temperature. Yet there are some molecules with strong enough intermolecular forces to form crystalline solids at or just below room temperature.
Ice is an example of a molecule that forms a crystalline solid. The lattice points of the three-dimensional structure are occupied by water molecules. This type of solid is a molecular solid. The attractions that hold the molecules in place are intermolecular forces, primarily
hydrogen bonding. The hydrogen of one molecule attracts a lone pair of an oxygen atom on an adjacent molecule.
Image Credit (ice):
Wikimedia Commons
Sucrose (table sugar) is another molecule that crystallizes to form a molecular solid. The sucrose molecules occupy the lattice points of the crystal. Sucrose is a large molecule with several O-H bonds as shown in the diagram below. Its large size contributes to the strength of the
London dispersion forces between nearby molecules. And the presence of the O-H bonds means that there will be strong
hydrogen bonding forces between molecules. These
intermolecular forces -
London dispersion and
hydrogen bonding - hold sucrose molecules in the lattice.
Image (sucrose):
Public Domain
We have often mentioned in this Chemistry Tutorial the seven elements that occur naturally as diatomic molecules (
remember Dr. HONClBrIF). Iodine is the one diatomic element that is a solid at room temperature. Its appearance is very crystalline in nature. Its molar mass and its size are the largest of all the diatomic molecules. The London dispersion forces between molecules are thus strong enough to hold I
2 molecules together in a crystalline solid. The lattice points of the solid are I
2 molecules. Iodine is another example of a molecular solid.
Photo Credits:
Iodine Crystals:
Wikimedia Commons
Space Filling Model:
Public Domain
Ball-and-Stick Model:
Public Domain
In addition to the seven diatomic elements, there are two additional elements that exist naturally as polyatomic molecules. These elements are sulfur (most commonly found as S
8) and phosphorus (occasionally found as P
4). Both nonmetals are solids at room temperature and form molecular solids. Their large mass and size leads to strong enough London dispersion forces that keep the molecules in the crystal lattice structure.
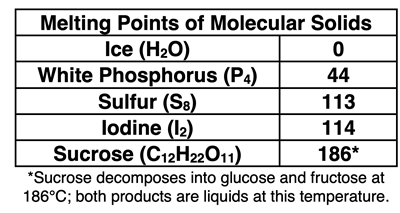
In each of our examples of molecular solids, there are molecules at the lattice positions of the crystal structure. The atoms of the molecules are held together by covalent bonds. But the molecules themselves are held together in a crystal lattice by intermolecular forces such as London dispersion forces and hydrogen bonding. As a group, these molecular solids have a low melting point relative to other crystalline solids. The relatively low melting point is attributed to the fact that the forces between molecules are considerably weaker than they are in other crystalline solids.
Network Solids
A network solid is a type of atomic solid. The fundamental particle present at the lattice positions is an atom of a nonmetal or metalloid. The atoms are held together by covalent bonds to form an organized three-dimensional array of atoms. These strong covalent bonds give network solids high melting points.
Carbon is an element that has many allotropes.
Allotropes are multiple physical forms of the same element. Two of the more common allotropes of carbon are graphite and diamond. Both are crystalline solids that have an atom as its fundamental particle. They differ from one another by their lattice structure.
In diamond, each carbon atom forms a covalent bond with four other carbon atoms. The carbon atom hybridizes with sp
3 orbitals and the four adjoining atoms form a tetrahedral around the carbon. The strength of the covalent bonds holding diamond in a three-dimensional array gives diamond its standing as one of the hardest materials on the block. Diamond is commonly used as a cutting and engraving tool. The strongly bonded 3D array also explains its high melting point of 4500°C.
The graphite form of carbon is quite different than diamond. The carbon atoms in graphite form a pattern of adjoining hexagons, arranged in flat, parallel layers. Each carbon atom hybridizes with sp
2 orbitals and bonds covalently with three other carbon atoms in the same layer to form a hexagon. The unhybridized p orbitals that are perpendicular to the hexagonal plane share delocalized electrons through pi-overlap to further strengthen the structure of the layer. Adjacent layers are held together by considerably weaker London dispersion forces; a small
sideways force on a layer will cause it to slide past the layer below it. Because of these weak attractions between layers and tendency for layers to slide past one another, graphite is often used as a lubricant. It is also used in pencils. The next time you swipe a layer of graphite off your pencil onto a page of paper, think
Chemistry for Better Living. The melting point of graphite is 3600°C.
Image Source:
Wikimedia Commons
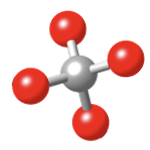
Quartz is our final example of a network solid. Quartz is composed of silicon and oxygen atoms in a 1:2 ratio. We would refer to its formula as SiO
2. Quartz is unique in that there are two different types of atoms at the lattice positions - silicon and oxygen atoms. Each silicon atom is bonded covalently to four oxygen atoms to form a tetrahedron with oxygen at the corners. And each of these oxygen atoms is bonded to a silicon atom of another tetrahedron, thus forming bridges between the tetrahedra. The result is a 3-dimensional network of strongly connected tetrahedron.
Quartz should not be thought of as a molecule of SiO
2. There is in fact no such molecular unit found in a crystal of quartz. In fact, it would be more accurate to think of a crystal of quartz as being a single, large molecule with an empirical formula (simplest ratio of atoms) of SiO
2. The bonds that hold the atoms together are covalent bonds. Their strength gives quartz a melting point of ~1660°C.
Metallic Solids
Metals are known for being good conductors of electricity and heat, for being ductile and malleable, and for having relatively high melting points. These properties are attributed to the structure and bonding assumed by metals. Metals are atomic solids. Metal atoms occupy the lattice points. But they are quite different than other crystalline solids because of their unique bonding habits.
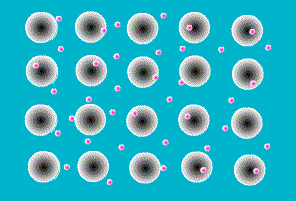
One of the simplest models to explain what holds metal atoms together in a crystal lattice is the
electron sea model. The electron sea model proposes that the outer shell of valence electrons in metal atoms are free to move from atom to atom. Rather than being bound to a specific atom, these outer shell electrons are delocalized about the entire sample. The model pictures a metal as a three-dimensional lattice of positively charged metal cations immersed in a sea of delocalized electrons. The attraction of metal cations for this sea of electrons provides the force that holds atoms in place.
The electron sea model explains many of the properties of metals. The delocalized nature of the electrons explains the electrical and thermal conductivity of metals. The massive sea of electrons in which the atoms are immersed allows atoms to slide past each other while maintaining an attraction to the electronic sea . This explains their malleability (capable of changing shape when
hammered) and ductility (capable of being extruded into wires). And the strong forces of attraction between the metal cations and the sea of electrons explains the high melting points of metals.
Ionic Solids
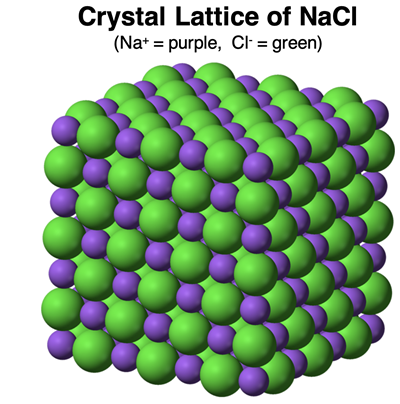
A sample of an ionic compound does not contain any molecules. That is to say, the cation and anion exists as separate ions located at the lattice points of a crystal structure. For instance, a sodium chloride (NaCl) sample does not consist of NaCl molecules. It consists of Na
+ and Cl
- ions forming an orderly and regular pattern within the lattice. Each Na
+ ion has six neighboring Cl
- ions. And each Cl
- ion has six neighboring Na
+ ions. There are no molecules … just a giant 3D grid of alternating positive and negative ions.
Instead of thinking in terms of molecules, we think in terms of formula units. Like an empirical formula, a
formula unit indicates the lowest whole number ratio of ions represented within a sample of an ionic compound. The formula unit for sodium chloride would be NaCl since the sodium ion and chloride ion are present in a 1:1 ratio. The formula unit for aluminum fluoride is AlF
3 since the aluminum ion and the fluoride ion are present with a 1:3 ratio. And the formula unit for aluminum oxide is Al
2O
3 since the aluminum ion and the oxide ion are present with a 2:3 ratio. The subscripts in the formula units represent the number of ions present in the ion ratio. These ions are held together by ionic bonds - attractions between oppositely charged ions.
Ionic bonds are considerably stronger than the intermolecular forces discussed in Lesson 1. This explains one of the identifying properties of ionic solids - high melting points. There are other properties unique to ionic solids. While they do not conduct electricity in the solid state, they do conduct electricity when dissolved or melted. When dissolved or melted, the ions are freed from the crystal lattice and can move more freely in order to conduct electricity. Several other properties were discussed in great detail
in Chapter 4 or our
Chemistry Tutorial.
We will discuss amorphous or non-crystalline solids in
the next page of Lesson 3. But before you fast forward head to that page, consider the following suggestions …
Before You Leave
- Download our Study Card on Crystalline and Amorphous Solids. Save it to a safe location and use it as a review tool. (Coming Soon.)
- The Check Your Understanding section below include questions with answers and explanations. It provides a great chance to self-assess your understanding.
Check Your Understanding
Use the following questions to assess your understanding. Tap the Check Answer buttons when ready.
1. Classify the following solis as being ionic, molecular, network, or metallic soids:
- Dry Ice (CO2)
- Copper
- Silicon (Si)
- FeCl3
2. Why are the melting points of molecular solids so much lower than the melting points of network solids?